Pseudocholinesterase Deficiency: Anesthesia Considerations
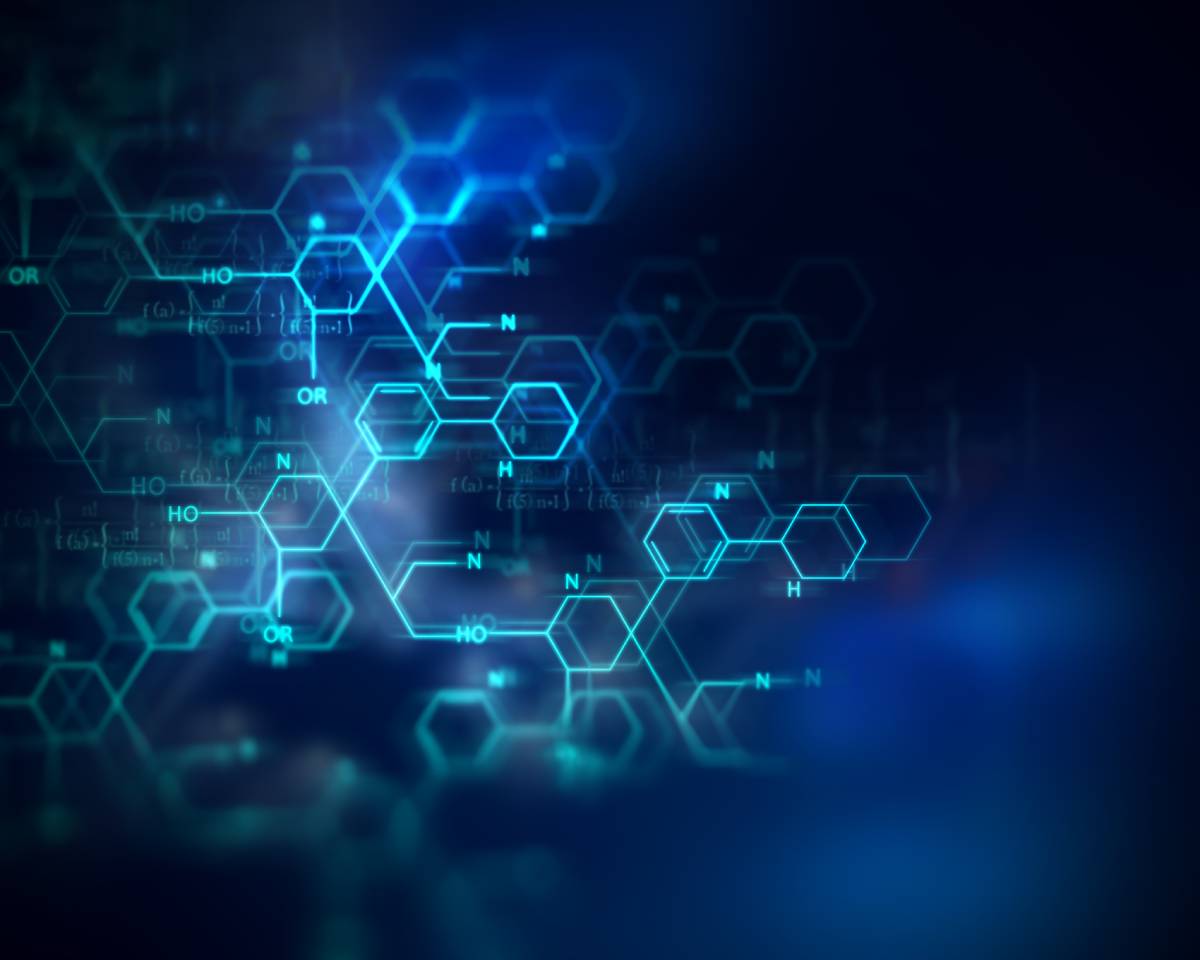
Pseudocholinesterase is an enzyme produced in the liver that is found in most tissues.i Also known as butyrylcholinesterase and plasma cholinesterase, the enzyme metabolizes choline esters, a class of molecules that act as neuromuscular blocking agents.ii The choline esters succinylcholine and mivacurium are commonly administered in conjunction with tracheal intubation and are normally metabolized relatively quickly by pseudocholinesterase. However, for those with pseudocholinesterase deficiency, a rare genetic disorder, these medications can result in prolonged neuromuscular paralysis and apnea, necessitating careful consideration from anesthesia providers.
Pseudocholinesterase deficiency is characterized by the reduced ability to metabolize and thereby terminate the effects of certain muscle relaxants, resulting in prolonged muscular paralysis from standard doses.iii Since pseudocholinesterase deficiency poses no other health risks, those who are deficient may only discover so once treated with succinylcholine or mivacurium. Treatment for a patient with this condition who is exposed to one of these choline esters includes mechanical ventilation until the effects of the neuromuscular blocking agent resolve spontaneously.iv While no cure exists for pseudocholinesterase deficiency, various interventions in cases of exposure can successfully reverse paralysis and apnea: these include administration of whole blood and the use of human serum cholinesterase.v Pseudocholinesterase individuals in need of anesthesia can avoid paralysis by undergoing treatment with different neuromuscular blockers, such as atracurium and rocuronium.vi
Pseudocholinesterase deficiency is an autosomal recessive genetic condition with many associated variants that can confer some degree of reduced choline ester metabolism. Homozygotes for variants of the BCHE gene, which provides instructions for pseudocholinesterase synthesis, present on average a prolonged response to succinylcholine compared to heterozygotes.vii The genetic basis for pseudocholinesterase deficiency was first identified in the 1950s, when it was discovered that families more sensitive to choline esters had less functional pseudocholinesterase.viii The dibucaine inhibition test was subsequently introduced, which measured the percent inhibition of pseudocholinesterase by dibucaine, a local anesthetic, and dibucaine resistance would eventually become the first discovered variant of pseudocholinesterase deficiency.ix Sodium fluoride has also been found to inhibit pseudocholinesterase activity, and inhibition via sodium fluoride was not correlated with dibucaine inhibition, indicating the existence of an additional genetic variant.9 Finally, there also exists a rare “silent variant” of the BCHE gene that results in the complete lack of pseudocholinesterase activity in homozygotes.x
Though rare, cases of acquired pseudocholinesterase deficiency have been documented. In one case, a patient became pseudocholinesterase deficient for 90 minutes after being treated with cyclophosphamide, a medication used for chemotherapy, several hours earlier; researchers were unsure of the pseudocholinesterase inhibition mechanism but advised caution in administering anesthesia after cyclophosphamide.xi More frequently, pre-existing medical conditions can impair pseudocholinesterase activity. Unsurprisingly, liver disease can limit pseudocholinesterase synthesis and normal production levels can be restored after a liver transplant.xii Reduced pseudocholinesterase activity has also been associated with renal disease,xiii malnutrition,xiv and various stages of pregnancy.xv
Considering the various genetic variants, medical conditions, and yet unknown molecular mechanisms that are linked to pseudocholinesterase deficiency and thereby bring about prolonged anesthesia-induced paralysis, anesthesia providers should take great care when prescribing choline ester anesthetics. Soliday et al.10 recommend the use of peripheral nerve stimulation to observe the metabolism of succinylcholine and recommend that providers suspect pseudocholinesterase deficiency if no significant response is detected after 15 minutes. Pseudocholinesterase deficiency is rare, but anesthesia providers should be equipped to detect it in advance and prevent its potentially harmful consequences.
References
i Andersson, M. L., et al. “Butyrylcholinesterase Deficiency and Its Clinical Importance in Anaesthesia: a Systematic Review.” Anaesthesia, vol. 74, no. 4, 2019, pp. 518–528., doi:10.1111/anae.14545.
ii Delacour, H., et al. “Butyrylcholinesterase Deficiency.” Annales De Biologie Clinique, vol. 74, no. 3, 31 May 2016, pp. 279–285.
iii Trujlio, R., and W. P. West. Pseudocholinesterase Deficiency. StatPearls Publishing, 2020.
iv Zhou, W., and L. Sheng “Delayed Recovery from Paralysis Associated with Plasma Cholinesterase Deficiency.” SpringerPlus, vol. 5, no. 1, 2016, doi:10.1186/s40064-016-3561-y.
v Lovely M. J., Patteson S. K., Beuerlein F. J., and J. T. Chesney. Perioperative blood transfusion may conceal atypical pseudocholinesterase. Anesth.Analg. vol 70., no. 3, 1990, pp. 326-327.
vi Lee, S., et al. “Butyrylcholinesterase Deficiency Identified by Preoperative Patient Interview.” Korean Journal of Anesthesiology, vol. 65, no. 6 Suppl, 2013.
vii Kalow, W., and K. Genest. “A Method For The Detection Of Atypical Forms Of Human Serum Cholinesterase. Determination Of Dibucaine Numbers.” Canadian Journal of Biochemistry and Physiology, vol. 35, no. 6, 1957, pp. 339-346.
viii Kalow, W., and N. Staron. “On Distribution And Inheritance Of Atypical Forms Of Human Serum Cholinesterase, As Indicated By Dibucaine Numbers.” Canadian Journal of Biochemistry and Physiology, vol. 35, no. 1, 1957, pp. 1305-1320.
ix Harris, H, and M. Whittaker. “Differential inhibition of human serum cholinesterase with fluoride: recognition of two new phenotypes.” Nature, vol. 191, 1961, pp. 496-498
x Soliday, F., et al. “Pseudocholinesterase Deficiency: A Comprehensive Review of Genetic, Acquired, and Drug Influences.” AANA Journal, vol. 78, no. 4, 2010, pp. 313-20.
xi Koseoglu, V., et al. “Acquired Pseudocholinesterase Deficiency after High-Dose Cyclophosphamide.” Bone Marrow Transplantation, vol. 24, 1999, pp. 1367–1368.
xii Aldrete, J. A., et al. “Changes Of Plasma Cholinesterase Activity During Orthotopic Liver Transplantation In Man.” Transplantation, vol. 23, no. 5, 1977, pp. 404–406., doi:10.1097/00007890-197705000-00004.
xiii Ryan, D. W., et al. “Postoperative serum cholinesterase activity following successful renal transplantation.” British Journal ofAnesthesiology, vol. 51, no. 9, 1979, pp. 881-884.
xiv Barclay, G. P., et al. “Pseudocholinesterase activity as a guide to prognosis in malnutrition.” American Journal of Clinical Pathology, vol. 59, no. 5, 1973, pp. 712-716.
xv Robertson, G., et al. “Serum cholinesterase deficiency: II. Pregnancy.” British Journal of Anesthesiology, vol. 38, no. 5, 1966, pp. 361-369.